How to make oxygen on the moon
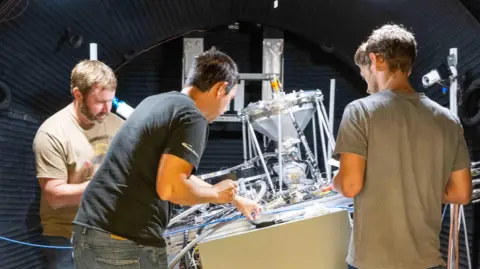
Inside a giant sphere, the engineers pored over their equipment. Before them stood a silvery metal contraption swathed in colourful wires – a box that they hope will one day make oxygen on the moon.
Once the team vacated the sphere, the experiment began. The box-like machine was now ingesting small quantities of a dusty regolith – a mixture of dust and sharp grit with a chemical composition mimicking real lunar soil.
Soon, that regolith was gloop. A layer of it heated to temperatures above 1,650C. And, with the addition of some reactants, oxygen-containing molecules began to bubble out.
“We’ve tested everything we can on Earth now,” says Brant White, a program manager at Sierra Space, a private company. “The next step is going to the moon.”
Sierra Space’s experiment unfolded at Nasa’s Johnson Space Center this summer. It is far from the only such technology that researchers are working on, as they develop systems that could supply astronauts living on a future lunar base.
Those astronauts will need oxygen to breathe but also to make rocket fuel for spacecraft that might launch from the moon and head to destinations further afield – including Mars.
Lunar base inhabitants might also require metal and they could even harvest this from the dusty grey debris that litters the lunar surface.
Much depends on whether we can build reactors able to extract such resources effectively or not.
“It could save billions of dollars from mission costs,” says Mr White as he explains that the alternative – bringing lots of oxygen and spare metal to the moon from Earth – would be arduous and expensive.
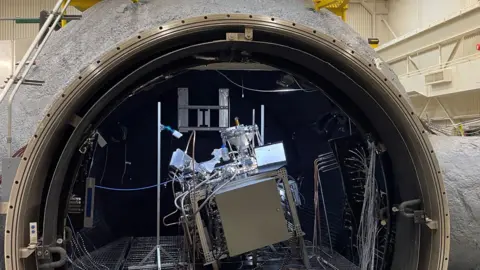
Luckily, the lunar regolith is full of metal oxides. But while the science of extracting oxygen from metal oxides, for example, is well understood on Earth, doing this on the moon is much harder. Not least because of the conditions.
The huge spherical chamber that hosted Sierra Space’s tests in July and August this year induced a vacuum and also simulated lunar temperatures and pressures.
The company says it has had to improve how the machine works over time so that it can better cope with the extremely jagged, abrasive texture of the regolith itself. “It gets everywhere, wears out all sorts of mechanisms,” says Mr White.
And the one, crucial, thing that you can’t test on Earth or even in orbit around our planet, is lunar gravity – which is roughly one sixth that of the Earth. It might not be until 2028 or later that Sierra Space can test its system on the moon, using real regolith in low gravity conditions.
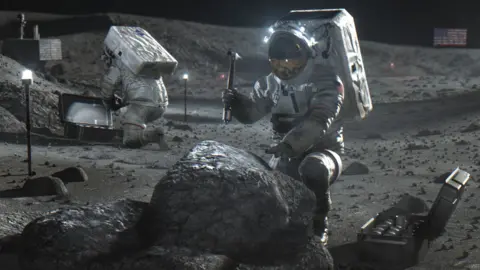
The moon’s gravity could be a real problem for some oxygen-extracting technologies unless engineers design for it, says Paul Burke at Johns Hopkins University.
In April, he and colleagues published a paper detailing the results of computer simulations that showed how a different oxygen-extracting process might be hindered by the moon’s relatively feeble gravitational pull. The process under investigation here was molten regolith electrolysis, which involves using electricity to split lunar minerals containing oxygen, in order to extract the oxygen directly.
The problem is that such technology works by forming bubbles of oxygen on the surface of electrodes deep within the molten regolith itself. “It is the consistency of, say, honey. It is very, very viscous,” says Dr Burke.
“Those bubbles aren’t going to rise as fast – and may actually be delayed from detaching from the electrodes.”
There could be ways around this. One could be to vibrate the oxygen-making machine device, which might jiggle the bubbles free.
And extra-smooth electrodes might make it easier for the oxygen bubbles to detach. Dr Burke and his colleagues are now working on ideas like this.
Sierra Space’s technology, a carbothermal process, is different. In their case, when oxygen-containing bubbles form in the regolith, they do so freely, rather than on the surface of an electrode. It means there is less chance of them getting stuck, says Mr White.
Stressing the value of oxygen for future lunar expeditions, Dr Burke estimates that, per day, an astronaut would require the amount of oxygen contained in roughly two or three kilograms of regolith, depending on that astronaut’s fitness and activity levels.
However, a lunar base’s life support systems would likely recycle oxygen breathed out by astronauts. If so, it wouldn’t be necessary to process quite as much regolith just to keep the lunar residents alive.
The real use case for oxygen-extracting technologies, adds Dr Burke, is in providing the oxidiser for rocket fuels, which could enable ambitious space exploration.
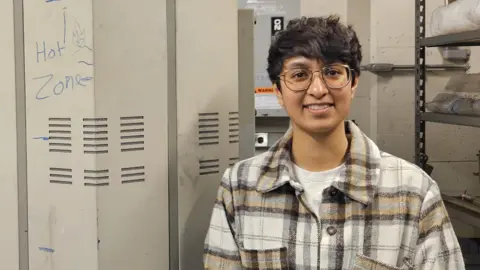
Obviously the more resources that can be made on the moon the better.
Sierra Space’s system does require the addition of some carbon, though the firm says it can recycle most of this after each oxygen-producing cycle.
Along with colleagues, Palak Patel, a PhD student at the Massachusetts Institute of Technology, came up with an experimental molten regolith electrolysis system, for extracting oxygen and metal from the lunar soil.
“We’re really looking at it from the standpoint of, ‘Let’s try to minimise the number of resupply missions’,” she says.
When designing their system, Ms Patel and her colleagues addressed the problem described by Dr Burke: that low gravity could impede the detachment of oxygen bubbles that form on electrodes. To counter this, they used a “sonicator”, which blasts the bubbles with sound waves in order to dislodge them.
Ms Patel says that future resource-extracting machines on the moon could derive iron, titanium or lithium from regolith, for example. These materials might help lunar-dwelling astronauts make 3D-printed spare parts for their moon base or replacement components for damaged spacecraft.
The usefulness of lunar regolith does not stop there. Ms Patel notes that, in separate experiments, she has melted simulated regolith into a tough, dark, glass-like material.
She and colleagues worked out how to turn this substance into strong, hollow bricks, which could be useful for building structures on the moon – an imposing black monolith, say. Why not?